Without any doubt the most innovative of
the current crop of proposals for high speed microwave networking, Angel
Technologies' HALO system could prove to be a serious competitor to the
emerging LEO satellite technology base.
The HALO system is based upon the idea of lifting a
communications payload analogous to that carried by a satellite to a
stratospheric station above a metropolitan area. The means of lifting
the payload is a stratospheric long endurance aircraft, in concept
similar to the U-2 of early sixties fame. Instead of pointing their
antennas at a satellite in orbit, subscribers point their antennas at a
patch of sky above the city, where the aircraft flies in a continuous
circular pattern, or orbit.
In effect the HALO system provides a metropolitan area
with its own private "pseudo-satellite", sitting at 50,000 ft rather
than the hundreds, thousands or tens of thousands of kilometres
characteristic of a real satellite.
The idea of an airborne microwave network node is
unconventional, but by no means unique. The US Air Force and DARPA are
developing a similar concept, called the Airborne Communications Node
(ACN), to be carried by the RQ-4A Global Hawk unmanned aircraft,
designed originally as a supplement or replacement for the Lockheed U-2
in the reconnaissance role, for which the U-2 is famous. DARPA's ACN
payload is currently in development and expected to be flown in the next
year or two. If its performance proves adequate, it would be fielded as
a supplement to satellites to support air, land and sea based
communications systems.
NASA are working on the ERAST program, which is aimed at
developing unmanned, long endurance stratospheric aircraft to supplement
or replace remote sensing satellites. The use of these aircraft to carry
a communications payload is also being explored. The most remarkable
product to date of the ERAST program is the massive solar cell powered
Pathfinder, and its successor, the 250 ft span Centurion. NASA's
intention is to explore the practicality of an unmanned robot aircraft
capable of remaining aloft for days and ultimately months at a time, and
flying at altitudes as high as 100,000 ft. The typical airliner seldom
flies above 40,000 ft. Interestingly, the biggest challenge in the ERAST
program has proven to be that of producing an energy storage system of
suitable performance, since once the sun goes down there is nothing to
feed the solar cells with. The strategy being pursued at this time are
fuel cells, which are fed from a storage device which is charged by the
solar cells.
There is clearly much activity under way at this time to
develop airframes capable of carrying a communications payload into the
stratosphere, so the obvious question the uninitiated will ask is why ?
Stratospheric vs
Orbital, Aircraft vs Satellites
When Arthur C. Clarke devised the geosynchronous earth
orbit (GEO) satellite five decades ago, little did he anticipate how
much this technology would change the world around us. Today the planet
is ringed by GEO satellites, packed very densely over areas of high
population density like the US and Europe.
By the sixties the Russians perfected the polar medium
earth orbit (MEO) Molniya satellites, and in the nineties we have
Iridium and Teledesic battling over who will own low earth orbit (LEO)
and the rest of MEO.
With the satellite market still showing strong growth in
broadcast applications and the impending foray by Teledesic into digital
interactive applications, it might seem curious that airborne
alternatives are being developed. The reasons are however trivially
simple to the microwave engineer - latency and path loss.
Latency is the time elapsed between a signal departing a
transmit antenna on the earth's surface, propagating up to the
satellite, passing through the satellite's hardware, departing the
satellite's transmit antenna, propagating back down to earth, and
finally impinging upon the earth-bound receive antenna.
For a GEO satellite latency can be as great as 250
milliseconds (or more), for an MEO satellite of the order of 50-100
milliseconds and for an LEO satellite of the order of 10 milliseconds.
Such delays are irrelevant if you are broadcasting TV or radio, but they
can cause some difficulty with digital data, especially where protocol
state machines become involved. Indeed GEO satcom links have been known
to cause serious havoc with protocols designed for landlines and unable
to accommodate a 1/2 second round trip delay.
Path loss is an equally painful problem with satellites.
Disregarding the effects of the lower atmosphere, which we will discuss
later, we have to grapple with the reality that the power level into our
receiver declines with the inverse square of distance from the
transmitter. The further we have to go, the bigger the antennas and the
more power we have to push out to achieve a sought bit rate over the
channel. Bigger antennas and more power translate directly into costs,
at the satellite end and at the ground station end of the link, if we
want serious bandwidth. Therefore low cost and high bit rates are
mutually exclusive in the satellite game.
Sending microwaves through the lower atmosphere however
causes further pain to the satellite system designer, since the lowest
layer of the atmosphere, the troposphere, is laden with free water
vapour, water droplets as cloud and rain, and gaseous oxygen. If we wish
to operate in the less congested centimetric bands, this tropospheric
"soup" will soak up our signal very rapidly. Rain and dense clouds are
particularly problematic.
If the satellite is directly overhead the distance
through this soup is minimal, but if the satellite's position in the sky
is moved away from the zenith, things get increasingly worse. This has
been one of the big selling points of LEO satellites compared to GEO
satellites, in that within the heavily populated temperate zones of the
earth your GEO satellite dish is at a disadvantage, operating at a very
shallow elevation angle.
If we are bouncing our microwave signals off a payload
on a stratospheric aircraft, things change dramatically. The distance
between our antenna shrinks from thousands or tens of thousands of
kilometers to mere tens of kilometres, with elevation angles typically
above 20 degrees. As a result the distance and thus latency drop by a
factor of between 25 and 1000 compared to LEO and GEO satellites
respectively. No less importantly pathlength loss drops dramatically
because of the inverse square law relationship, and long paths through
the tropospheric soup are minimised. As a result you can achieve a very
high bit rate with a fraction of the transmitted power and antenna size
required to achieve the same when bouncing off a satellite.
The physics of microwave propagation and Shannon's law
of channel capacity give the airborne system an unbeatable advantage
over the satellite.
Aircraft have other, more pragmatic advantages over
satellites. They are much cheaper to build and operate, in comparison
with satellite manufacture (read hand crafting) and lofting into orbit.
Airborne communications payloads can also be designed to a much cheaper
commercial avionic standard, since they can be repaired easily on the
ground, a choice not available to a satellite operator. The atmosphere
shields much of the radiation which can damage satellites and degrade
their semiconductor electronics over time thereby contributing to a
greater operational life for the electronics.
In practical terms, an airborne package can be
maintained in service indefinitely by ongoing repairs, preventive
maintenance, and can be upgraded and modified at any time. Those who may
doubt this should consider that the B-52s intended to be in service in
2030 were built in the early sixties. Many airliners in service today
were built in the fifties and sixties.
Should a satellite be lost due failure, getting a
replacement into orbit can take up to years. With aircraft, a spare may
be airborne in minutes if it is fuelled and ready.
What are the limitations of aircraft compared to
satellites ? The first and foremost, is that they are quite limited in
footprint and if we set a 20 degree ground station elevation angle as a
limit, can reach out to a distance of about three times their operating
altitude. To cover a radius of 100 km the aircraft needs to be at about
100,000 ft altitude. At a 50-60 km radius we get an altitude of about
50,000-60,000 ft, which is the domain in which the HALO and DARPA ACN
systems are intended to operate.
The second limitation of aircraft is endurance, unlike a
satellite which hangs in orbit, an aircraft needs to be fed on energy to
remain aloft. Excluding the NASA ERAST project, this means hundreds or
thousands of litres of kerosene to remain up for a decent number of
hours.
Addressing the requirements of stratospheric operating
altitude and long endurance, such as 8, 12, 16, 24 hours or longer, we
end up with a aircraft not unlike the U-2 which is the progenitor of the
species - very large wings, and very light structure. Such aircraft are
finicky to fly and handle on the ground.
As a result an aircraft capable of replacing a satellite
will be limited in terms of the severity of weather it can handle on
takeoff or landing, and if 45 knot gusting winds hit its home base
airfield, it will have to elsewhere to refuel.
Unlike satellites, aircraft require at this time pilots
to fly them, although current trends suggest that unmanned operation may
become more commonplace in time. As yet civil air traffic control has
yet to catch up with the military in terms of techniques for handling
robot aeroplanes in controlled airspace.
Providing an aircraft can be built which has the
aerodynamic efficiency to sustain flight for 8-12 hours in the
stratosphere with a decently sized communications payload, then the
advantages of an airborne system over a satellite can be realised. The
HALO system is the first commercial attempt to do so.

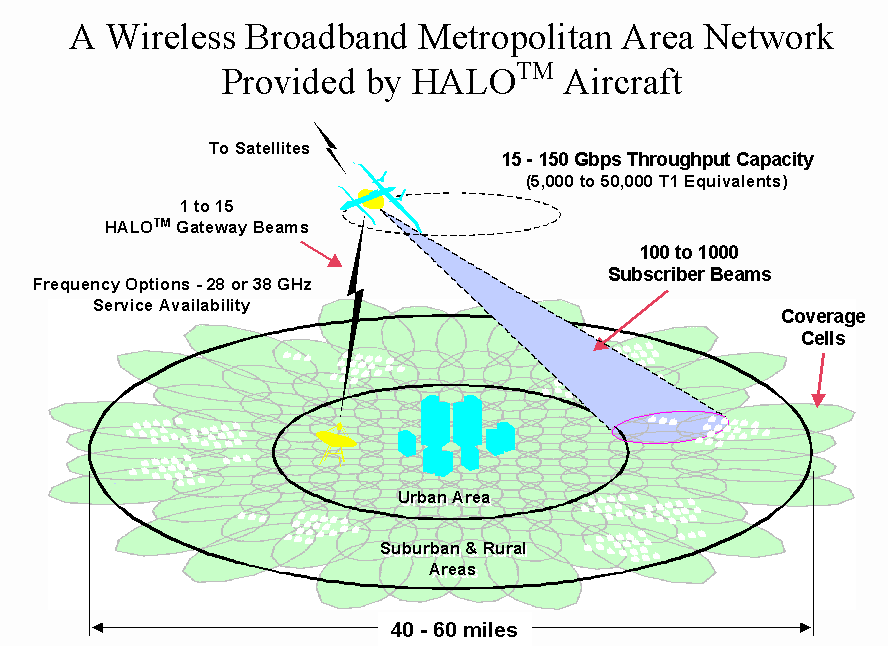
The Proteus "carrier" aircraft for the HALO system was
designed and built by Burt Rutan's Scaled Composites in Mojave, the same
company which built the record breaking Voyager aircraft which
circumnavigated the globe non-stop. The Proteus is largely built with
composite materials, and has a gross weight of up to 6.4 tonnes, of
which up to 2.8 tonnes is kerosene to fuel the pair of Williams FJ44-2E
jet engines. On station endurance is up to 12 hours. Seating is
available for two pilots, with an extra seat for a relief pilot or
passenger, the cabin is pressurised and high altitude pressure suits are
not required. The design station altitude is between 52,000 and 64,000
ft, well above airliner cruise altitudes.
The HALO communications payload is intended to operate
in two 300 MHz slices of the 28 GHz band, centred on 27.65 GHz and 28.2
GHz, with a 250 MHz "guard channel" for supporting services at 27.95
GHz. Each of the up to 100 coverage cells would have access to one or
more duplex OC-1 channels of 51.84 Mbits/s capacity and 40 MHz
bandwidth, which yields an aggregate capacity of the order of about 5
Gbit/s.



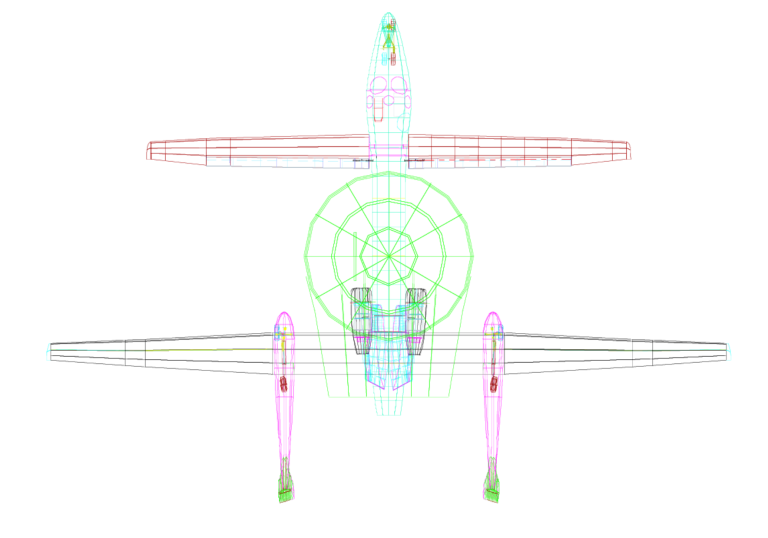
Angel technologies claim that a suitable HALO payload
could concurrently support between 10,000 and 75,000 1.5 Mbit/s T1
symmetrical channels within a footprint of up to 96 km diameter, making
some assumptions about frequency reuse and subscriber load sharing.
The baseline pod architecture described by Angel
Technologies is based upon a central high speed digital switch, tied to
an array of SONET multiplexers, each of which in turn feeds into a 28
GHz band communications package and antenna.
The subscriber equipment package is relatively simple,
comprising a Radio Frequency Unit and a Network Interface Unit. The
Radio Frequency Unit contains the steerable dish antenna, intended to be
about 0.3 metres in diameter, a millimetric band transmitter of between
100 mW and 1 Watt output power, a millimetric band receiver, and the
antenna steering mechanism. Because the aircraft is orbiting at a radius
of up to 7.5 km, even at the extremities of coverage the subscriber
antenna will have to be steered by a servo loop to maintain track.
However, the angular range required is very modest and about several
degrees of arc, and the angular rate very slow. Therefore the motion of
the antenna will be minimal.
The Network Interface Unit contains the modulators and
demodulators for the signal, and the feed to and from the Radio
Frequency Unit is done in the 1.4 GHz band, with up/down conversion
performed in the Radio Frequency Unit. It is intended that existing 28
GHz terrestrial networking equipment be adapted for this purpose by the
addition of antenna tracking hardware and software.
The intent is to provide the user with a duplex 51.84
Mbits/s capacity channel via the Network Interface Unit to a local on
site LAN.
Given the use of a central high speed switch in the HALO
pod, the system results in a star topology network with a propagation
latency between subscribers at the extremities of coverage of about 0.3
milliseconds.
Intended customers for the HALO system include ISPs,
corporate users, government users and individual subscribers with a
strong demand for bandwidth.
Analysis and
Critique
The HALO system has yet to be proven operationally and
since it is the first of its kind, there are few parallels against which
it can be compared. The idea is radical and innovative, and thus is
likely to attract much scepticism if not criticism.
The operational issues in maintaining an aircraft
continuously on station are well understood and have been practiced by
military aviators for many decades. Plenty of spare parts, spare pilots
and ample maintenance personnel can provide for virtually 100%
operational uptime. Of course profitability can be impaired to the
operator if too much spare hardware and too many spare personnel are
required. Given the exceptional service reliability in the airline
industry, and the pool of former military pilots in the commercial
aviation work-force, this is not a problem area. If an operator has
difficulties, it is a problem easily solved.
The technology required for the design and construction
of the HALO pod is well established and in many instances very mature.
Adapting it for an airborne application will require primarily
improvements to the cooling systems to accommodate the very low
atmospheric density at the operating altitude. This too is an area which
is well understood, since much commercial and military avionic equipment
exists which has been designed to operate under such conditions. We can
assume that the pod will contain an internal environmental control
system (ECS) which will be driven either by the aircraft's generators or
bleed air from the engines. Therefore, excluding any engineering
bloopers during design, there are no fundamental reasons why this aspect
of the system cannot be implemented very robustly.
The ground station equipment is likely to be derived
from existing off-the-shelf equipment and the requirement for an
additional antenna steering servo loop verges on the technically
trivial. Precision antenna tracking techniques were perfected in the
sixties and this is not a technologically difficult area.
Ground station visibility of the aircraft will require
that antennas be judiciously positioned, with a clear line of sight to
the aircraft's orbit. In inner city / CBD areas this will dictate roof
mounting. In suburban areas, an exterior wall mount under the roof may
be adequate, providing no trees or other foliage obscure the line of
sight.
The only genuine criticism I can level at the system
design is that the power budgets published by Angel Technologies fall
slightly short of what is required to achieve the intended 51.84 Mbits/s
channel capacity at maximum range. I simulated the propagation losses
using a very recently developed software package which accounted for
cloud, rain, oxygen and water vapour losses, and is much more accurate
than the standard tables commonly used in satcom design. At 28 GHz
oxygen and water vapour absorption can bite quite badly, at modest slant
ranges, and rain and dense cloud can be real show stoppers.
The practical consequence of this is that subscribers
may need to use antennas of 0.6 to 1 metre diameter, rather than 0.3
metre diameter, to punch through cloud and rain. How frequently such
weather conditions arise will depend on the geography of the city in
question.
In the context of Australia's underdeveloped and
overpriced subscriber level high speed data communications environment,
the HALO system looks most interesting. Since most Australian capitals
enjoy, by US standards, excellent weather conditions and frequently
excellent flying weather, there are no fundamental obstacles to
operating such a system in this country with very high availability.
Indeed the frequent hot and dry weather over much of the year in many of
our capitals would be optimal for the HALO system and possibly allow it
to operate over a larger footprint. HALO would cope far worse in
monsoonal areas where extreme humidity, dense cloud and heavy rainfall
are typical for a large part of the year and a major issue for
millimetric band operations.
How the HALO system would fit into our regulatory
framework is an interesting point to ponder, as would be the issue of
customer acceptance in a market which is frequently shy of new
technology.
Should Angel Technologies succeed and establish a
successful service in the US, they could become a serious commercial
threat to providers such as Teledesic, by displacing them from the
lucrative urban and CBD markets. Equally so HALO could damage portions
of the emerging ADSL/VDSL markets, by offering a highly capable
alternative. Since the basic design lends itself to incremental
upgrades, and the use of multiple aircraft to increase coverage
footprint and density, it does not suffer the inherently slow ramp up we
see in cabled systems.
The perspective view is that airborne networking is
about to emerge as a viable technological alternative to satellites, and
may produce some interesting long term changes in the market - at the
expense of established players.